Roles of Ionic Environments in Growth of Human Cancer Cell and Potentials of Ion Transporter Blockers in Cancer Therapies
Yoshinori Marunaka1-3*
1Department of Molecular Cell Physiology, Graduate School of Medical Science, Kyoto Prefectural University of Medicine, Kyoto, Japan
2Department of Bio-Ionomics, Graduate School of Medical Science, Kyoto Prefectural University of Medicine, Kyoto, Japan
3Japan Institute for Food Education and Health, Heian Jogakuin (St. Agnes’) University, Kyoto, Japan
- Corresponding Author:
- Yoshinori Marunaka
Department of Molecular Cell Physiology
Kyoto Prefectural University of Medicine
465 Kajii-cho, Kamigyoku, Kyoto 602-8566, Japan
Tel: +81-75-251-5311
Fax: +81-75-251-0295
E-mail: marunaka@koto.kpum.ac.jp
Received Date: December 09, 2013; Accepted Date: December 30, 2013; Published Date: January 08, 2014
Citation: Marunaka Y (2014) Roles of Ionic Environments in Growth of Human Cancer Cell and Potentials of Ion Transporter Blockers in Cancer Therapies. J Gastroint Dig Syst 3:163. doi: 10.4172/2161-069X.1000163
Copyright: © 2014 Marunaka Y. This is an open-access article distributed under the terms of the Creative Commons Attribution License, which permits unrestricted use, distribution, and reproduction in any medium, provided the original author and source are credited.
Visit for more related articles at Journal of Gastrointestinal & Digestive System
Introduction
Cancer cells live in hypoxic, hypo-nutrient circumstances provided by insufficient angiogenesis due to fast rates of cancer cell proliferation [1,2]. These circumstances lead cancer cells via production of ATP as energy sources mainly via high rate glycolysis, while most normal cells produce ATP via oxidation of pyruvate in mitochondria with relatively low-rate glycolysis compared with cancer cells [3]. The high-rate glycolys is forming lactate produces a large amount of H+, providing acidic micro environments around cancer cells [4]. Interestingly, cancer cells keep the cytosolic pH (pHc) slightly higher than that of normal cells even under these environmental conditions provided by a large amount of H+ due to high-rate glycolysis [5,6]. From a viewpoint of cell cycle arrest, apoptosis and cell growth, pHc of tumor cells kept at a level slightly higher than that of normal cells is a key factor for prevention from cell cycle arrest and apoptosis even under acidic microenvironments around cancer cells [7,8]. These findings clearly indicate that cancer cells have to maintain their pHc at a level slightly higher than that of normal cells for survival even under acidic conditions with production of a large amount of H+. For maintenance of pHc at a level slightly higher even under acidic environmental conditions than normal one, expression and/or activity of H+ and/or HCO3 -transporting systems in cancer cells would be expected to be higher than normal cells. Further, it is notable that cancer cells utilize a self-nutrient-recycling autophagy system for their survival even under starvation conditions [9-11]. The self-nutrient-recycling autophagy occurs in lysosome, and the intralysosomal pH is much lower than the cytosolic one [12,13]. Cl-also plays a key role in regulation of pHc and intra-lysosomal pH. In this article, I discuss roles of ionic circumstances in growth and lysosomal function in cancer cells.
Expression of ion transporters regulating pHc in cancer cells
Cancer cells have been reported to express four major ion transporters participating in keeping pHc at a normal or slightly higher level even under acidic environmental conditions than normal one [14- 16]. These four major ion transporters regulating pHc are classified into two categories [14-16]; i) H+ transporters, and ii) HCO3 -transporters. H+ transporters, Na+/H+ exchanger (NHE) and H+ pumps (V-type H+-ATPase, etc.), directly extrude H+from the cytosolic space to the extracellular or into the intra-lysosomal one keeping high pHc. On the one hand, HCO3 -transporters, Na+/HCO3 - cotransporter (NBC), Na+- driven Cl-/HCO3 - exchanger (NDCBE), and Cl-/HCO3 - exchanger (AE), participate in HCO3 - movement across the plasma membrane regulating pHc. Unlike H+ transporters these HCO3 -transporters do not participate in unidirectional transport of HCO3-; i.e., NBC and NDCBE contribute to uptake of HCO3 - into the cytosolic space using the electrochemical gradient of Na+ for elevation of pHc, but AE extrudes HCO3 - from the cytosolic space to the extracellular one for a decrease in pHc instead of elevation of the cytosolic Cl- concentration under general conditions.
Roles of NHE and its Inhibitor in Growth of Cancer Cells and Ionic Circumstances in Cancer Cells
Among the pHc-regulating ion transporters mentioned above, NHE is the major regulator of pHc ubiquitously expressed in most cells including cancer cells, and 10 isoforms of NHEs have been identified [17-21]. NHE1, an isoform of NHE, is the most ubiquitously expressed one among 10 isoforms [22-28]. Various types of growth factors, integlins, tyrosine phosphatases and cytokines regulate activity of NHE1 [22-28]. NHE, in particular NHE1, in cancer cells plays a crucial role in maintenance of pHc at a level slightly higher than normal one [29]. Proliferation and migration of many cancer cells require pHc slightly higher than normal one, which depends on NHE activity [19-21].Therefore, NHE inhibitors should be investigated as anticancer drugs inhibiting proliferation of cancer cells underacidic micro-environmental conditions. Indeed, many researchers have tried to investigate action of NHE inhibitors on proliferation of cancer cells in detail [30]. Recently, we [31] have also reported that 5-(N-ethyl- N-isopropyl) amiloride (EIPA, an NHE inhibitor [32]) inhibits the proliferation of human gastric cancer cells. It is generally thought that inhibition of NHE decreases pHc. However, our report [31] indicates that inhibition of NHE has no influence on pHc, but decreases the cytosolic Cl- concentration ([Cl-]c).The cytosolic Cl- has been reported to play important roles in various cell functions including proliferation of cancer cells [33-48]. The role of cytosolic Cl- in cancer cell proliferation has been also investigated; when [Cl-]c is experimentally decreased by lowering the extracellular Cl- concentration, gastric cancer cells show diminution of cell proliferation via a p53-independent p21- upregulation pathway [36,43]. Thus, the cytosolic Cl- is one of the key regulators in cancer cell proliferation [31] (Figure 1).
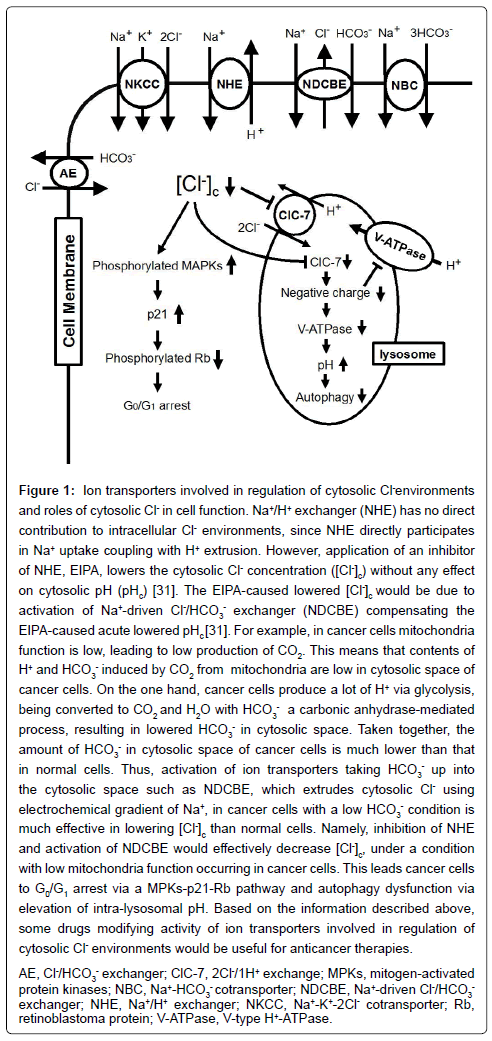
Figure 1: Ion transporters involved in regulation of cytosolic Cl- environments
and roles of cytosolic Cl- in cell function. Na+/H+ exchanger (NHE) has no direct
contribution to intracellular Cl- environments, since NHE directly participates
in Na+ uptake coupling with H+ extrusion. However, application of an inhibitor
of NHE, EIPA, lowers the cytosolic Cl- concentration ([Cl-]c) without any effect
on cytosolic pH (pHc) [31]. The EIPA-caused lowered [Cl-]c would be due to
activation of Na+-driven Cl-/HCO3 - exchanger (NDCBE) compensating the
EIPA-caused acute lowered pHc [31]. For example, in cancer cells mitochondria
function is low, leading to low production of CO2. This means that contents of
H+ and HCO3 - induced by CO2 from mitochondria are low in cytosolic space of
cancer cells. On the one hand, cancer cells produce a lot of H+ via glycolysis,
being converted to CO2 and H2O with HCO3 - a carbonic anhydrase-mediated
process, resulting in lowered HCO3 - in cytosolic space. Taken together, the
amount of HCO3 - in cytosolic space of cancer cells is much lower than that
in normal cells. Thus, activation of ion transporters taking HCO3 - up into
the cytosolic space such as NDCBE, which extrudes cytosolic Cl- using
electrochemical gradient of Na+, in cancer cells with a low HCO3 - condition is
much effective in lowering [Cl-]c than normal cells. Namely, inhibition of NHE
and activation of NDCBE would effectively decrease [Cl-]c, under a condition
with low mitochondria function occurring in cancer cells. This leads cancer cells
to G0/G1 arrest via a MPKs-p21-Rb pathway and autophagy dysfunction via
elevation of intra-lysosomal pH. Based on the information described above,
some drugs modifying activity of ion transporters involved in regulation of
cytosolic Cl- environments would be useful for anticancer therapies.
AE, Cl-/HCO3 - exchanger; ClC-7, 2Cl-/1H+ exchange; MPKs, mitogen-activated
protein kinases; NBC, Na+-HCO3 - cotransporter; NDCBE, Na+-driven Cl-/HCO3 -
exchanger; NHE, Na+/H+ exchanger; NKCC, Na+-K+-2Cl- cotransporter; Rb,
retinoblastoma protein; V-ATPase, V-type H+-ATPase.
Autophagy in Cancer Cells
Cells survive even under starvation conditions utilizinga selfnutrient- recycling autophagy system [9-11]. Cells check if they should degrade long-lived or miss-folded proteins, and damaged organelles such as mitochondria utilizing this autophagy system [49-51]. The autophagy system is induced by starvation-caused poverty of nutrients, mainly amino acids, for cells to survive utilizing reproduced nutrients originally contained in cells themselves [9]. Recycled amino acids reproduced by autophagy are utilized for new proteins synthesis [9]. Autophagy is generally activated by starvation, however autophagy process functions even under conditions with rich nutrition [52]. Activity of autophagy is closely correlated with not only cancer [53], but also other diseases such as diabetes mellitus [54], Parkinson disease [51], and inflammatory disease, Crohn disease [55].
Autophagy and Intra-Lysosomal Ionic Circumstances in Cancer Cells
Autophagy ability in cancer cells is much higher than normal cells, since cancer cells have to survive under hypoxic, hypo-nutrient micro environments utilizing recyclable nutrition such as amino acids [53]. It has been reported that Atg5 or Atg7 plays a key role in autophagy function; lack of Atg5 or Atg7 impairs the autophagy system and apoptosis in cancer cells [56-58]. Lysosomal machineries catabolize cell components, indicating that lysosome is a key organelle producing autophagy function in degradation of various compounds [11]. It is well known that the intra-lysosomal pH is lower than pHc [12,13], and that this lower intra-lysosomal pH is a key factor to maintain the digesting activity of lysosomal enzymes; i.e., lysosomal enzymes require low pH to maintain their enzymatic activities [59]. The V-type H+-ATPase (proton pump) generates the intra-lysosomal low pH cooperating with ClC-7located on the lysosome membrane [60,61]. ClC- 7has stoichiometry of 2Cl-/1H+ exchange, and is assumed to primarily behave as a Cl- permeation pathway across the lysosomal membrane [60], although there is still some contradictory observations (e.g., [62]). Mutation of ClC-7 impairs lysosomal function, which is detected as abnormal accumulation of proteins into the intra-lysosomal space [63], meaning that the function of ClC-7 as a Cl- permeation pathway would contribute to lysosomal function (protein degradation) via maintenance of low intra-lysosomal pH. Further, knock down of ClC- 7 impairs lysosomal acidification [60], and inhibits cell proliferation associated with abnormal accumulation of proteins in lysosomes [64]. These studies [60,63,64] suggest that ClC-7 would be essential as Clmovement/ transport for maintenance of low intra-lysosomal pH and autophagy-dependent cell proliferation. Although these studies [60,63,64] suggest the importance of Cl- movement/transport via ClC- 7, the importance of functional presence of Cl-is not confirmed. Our study suggests that the presence of Cl- itself plays an essential role in autophagy (Figure 1).
Conclusion
Some drugs modifying activity of ion transporters involved in cytosolic Cl- environments would be useful for anticancer therapies (Figure 1).
Acknowledgements
This work was supported by Grants-in-Aid from Japan Society of the Promotion of Science (25670111), Fuji Foundation for Protein, Research Conference for Cell Function, and The Salt Science Research Foundation (1235).
References
- Chiche J, Brahimi-Horn MC, Pouysségur J (2010) Tumour hypoxia induces a metabolic shift causing acidosis: a common feature in cancer. J Cell Mol Med 14: 771-794.
- Cui J, Gong Z, Shen HM (2013) The role of autophagy in liver cancer: molecular mechanisms and potential therapeutic targets. Biochim Biophys Acta 1836: 15-26.
- Gillies RJ, Robey I, Gatenby RA (2008) Causes and consequences of increased glucose metabolism of cancers. J Nucl Med 49 Suppl 2: 24S-42S.
- Parks SK, Chiche J, Pouysségur J (2013) Disrupting proton dynamics and energy metabolism for cancer therapy. Nat Rev Cancer 13: 611-623.
- Gillies RJ, Raghunand N, Garcia-Martin ML, Gatenby RA (2004) pH imaging. A review of pH measurement methods and applications in cancers. IEEE Eng Med Biol Mag 23: 57-64.
- Becelli R, Renzi G, Morello R, Altieri F (2007) Intracellular and extracellular tumor pH measurement in a series of patients with oral cancer. J Craniofac Surg 18: 1051-1054.
- Che XF, Akiyama S, Tomoda A (2008) Suppression of the proliferation of cancer cell lines, KB-3-1 and K562 cells preceded by a decrease in intracellular pH caused by phenoxazine derivatives. Oncol Rep 19: 1253-1258.
- De Milito A, Iessi E, Logozzi M, Lozupone F, Spada M, et al. (2007) Proton pump inhibitors induce apoptosis of human B-cell tumors through a caspase-independent mechanism involving reactive oxygen species. Cancer Res 67: 5408-5417.
- He C, Klionsky DJ (2009) Regulation mechanisms and signaling pathways of autophagy. Annu Rev Genet 43: 67-93.
- Kroemer G, Marino G, Levine B (2010) Autophagy and the integrated stress response. Mol Cell 40: 280-293
- Mizushima N, Komatsu M (2011) Autophagy: renovation of cells and tissues. Cell 147: 728-741.
- Riejngoud DJ, Tager JM (1973) Measurement of intralysosomal pH. Biochim Biophys Acta 297: 174-178.
- Ohkuma S, Poole B (1978) Fluorescence probe measurement of the intralysosomal pH in living cells and the perturbation of pH by various agents. Proc Natl Acad Sci U S A 75: 3327-3331.
- Hernández A, Serrano G, Herrera-Palau R, Pérez-Castiñeira JR, Serrano A (2010) Intraorganellar acidification by V-ATPases: a target in cell proliferation and cancer therapy. Recent Pat Anticancer Drug Discov 5: 88-98.
- Nishi T, Forgac M (2002) The vacuolar (H+)-ATPases--nature's most versatile proton pumps. Nat Rev Mol Cell Biol 3: 94-103.
- Parks SK, Chiche J, Pouyssegur J (2011) pH control mechanisms of tumor survival and growth. J Cell Physiol 226: 299-308.
- Lee SH, Kim T, Park ES, Yang S, Jeong D, et al. (2008) NHE10, an osteoclast-specific member of the Na+/H+ exchanger family, regulates osteoclast differentiation and survival [corrected]. Biochem Biophys Res Commun 369: 320-326.
- Malo ME, Fliegel L (2006) Physiological role and regulation of the Na+/H+ exchanger. Can J Physiol Pharmacol 84: 1081-1095.
- Friday E, Oliver R 3rd, Welbourne T, Turturro F (2007) Role of epidermal growth factor receptor (EGFR)-signaling versus cellular acidosis via Na+/H+ exchanger1(NHE1)-inhibition in troglitazone-induced growth arrest of breast cancer-derived cells MCF-7. Cell Physiol Biochem 20: 751-762.
- Koliakos G, Paletas K, Kaloyianni M (2008) NHE-1: a molecular target for signalling and cell matrix interactions. Connect Tissue Res 49: 157-161.
- Lee GH, Yan C, Shin SJ, Hong SC, Ahn T, et al. (2010) BAX inhibitor-1 enhances cancer metastasis by altering glucose metabolism and activating the sodium-hydrogen exchanger: the alteration of mitochondrial function. Oncogene 29: 2130-2141.
- Xue J, Zhou D, Yao H, Gavrialov O, McConnell MJ, et al. (2007) Novel functional interaction between Na+/H+ exchanger 1 and tyrosine phosphatase SHP-2. Am J Physiol Regul Integr Comp Physiol 292: R2406-2416.
- Fujiwara Y, Higuchi K, Takashima T, Hamaguchi M, Hayakawa T, et al. (2006) Roles of epidermal growth factor and Na+/H+ exchanger-1 in esophageal epithelial defense against acid-induced injury. Am J Physiol Gastrointest Liver Physiol 290: G665-673.
- Baldini PM, De Vito P, Vismara D, Bagni C, Zalfa F, et al. (2005) Atrial natriuretic peptide effects on intracellular pH changes and ROS production in HEPG2 cells: role of p38 MAPK and phospholipase D. Cell Physiol Biochem 15: 77-88.
- Chien EJ, Chang CP, Lee WF, Su TH, Wu CH (2006) Non-genomic immunosuppressive actions of progesterone inhibits PHA-induced alkalinization and activation in T cells. J Cell Biochem 99: 292-304.
- Goldman A, Shahidullah M, Goldman D, Khailova L, Watts G, et al. (2010) A novel mechanism of acid and bile acid-induced DNA damage involving Na+/H+ exchanger: implication for Barrett's oesophagus. Gut 59: 1606-1616.
- Lui KE, Panchal AS, Santhanagopal A, Dixon SJ, Bernier SM (2002) Epidermal growth factor stimulates proton efflux from chondrocytic cells. J Cell Physiol 192: 102-112.
- Coaxum SD, Garnovskaya MN, Gooz M, Baldys A, Raymond JR (2009) Epidermal growth factor activates Na(+/)H(+) exchanger in podocytes through a mechanism that involves Janus kinase and calmodulin. Biochim Biophys Acta 1793: 1174-1181.
- Gatenby RA, Smallbone K, Maini PK, Rose F, Averill J, et al. (2007) Cellular adaptations to hypoxia and acidosis during somatic evolution of breast cancer. Br J Cancer 97: 646-653.
- Li S, Bao P, Li Z, Ouyang H, Wu C, et al. (2009) Inhibition of proliferation and apoptosis induced by a Na+/H+ exchanger-1 (NHE-1) antisense gene on drug-resistant human small cell lung cancer cells. Oncol Rep 21: 1243-1249.
- Hosogi S, Miyazaki H, Nakajima K, Ashihara E, Niisato N, et al. (2012) An inhibitor of Na+/H+ exchanger (NHE), ethyl-isopropyl amiloride (EIPA), diminishes proliferation of MKN28 human gastric cancer cells by decreasing the cytosolic Cl- concentration via DIDS-sensitive pathways. Cell Physiol Biochem 30: 1241-1253.
- Masereel B, Pochet L, Laeckmann D (2003) An overview of inhibitors of Na(+)/H(+) exchanger. Eur J Med Chem 38: 547-554.
- Marunaka Y, Niisato N, O'Brodovich H, Eaton DC (1999) Regulation of an amiloride-sensitive Na+-permeable channel by a beta2-adrenergic agonist, cytosolic Ca2+ and Cl- in fetal rat alveolar epithelium. J Physiol 515 : 669-683.
- Kitagawa M, Niisato N, Hosogi S, Shiozaki A, Otsuji E, et al. (2013) A role of K+-Cl- cotransporter in the cell cycle regulation of breast cancer MDA-MB-231 cells J Physiol Sci 63: S137.
- Miyazaki H, Shiozaki A, Niisato N, Ohsawa R, Itoi H, et al. (2008) Chloride ions control the G1/S cell-cycle checkpoint by regulating the expression of p21 through a p53-independent pathway in human gastric cancer cells. Biochem Biophys Res Commun 366: 506-512.
- Nakajima K, Miyazaki H, Niisato N, Marunaka Y (2007) Essential role of NKCC1 in NGF-induced neurite outgrowth. Biochem Biophys Res Commun 359: 604-610.
- Nakajima K, Niisato N, Marunaka Y (2011) Quercetin stimulates NGF-induced neurite outgrowth in PC12 cells via activation of Na+/K+/2Cl- cotransporter. Cell Physiol Biochem 28: 147-156
- Nakajima K, Niisato N, Marunaka Y (2012) Enhancement of tubulin polymerization by Cl(-)-induced blockade of intrinsic GTPase. Biochem Biophys Res Commun 425: 225-229.
- Niisato N, Eaton DC, Marunaka Y (2004) Involvement of cytosolic Cl- in osmoregulation of alpha-ENaC gene expression. Am J Physiol Renal Physiol 287: F932-939.
- Niisato N, Ohta M, Eaton DC, Marunaka Y (2012) Hypotonic stress upregulates β- and γ-ENaC expression through suppression of ERK by inducing MKP-1. Am J Physiol Renal Physiol 303: F240-252.
- Niisato N, Taruno A, Marunaka Y (2007) Involvement of p38 MAPK in hypotonic stress-induced stimulation of beta- and gamma-ENaC expression in renal epithelium. Biochem Biophys Res Commun 358: 819-824.
- Ohsawa R, Miyazaki H, Niisato N, Shiozaki A, Iwasaki Y, et al. (2010) Intracellular chloride regulates cell proliferation through the activation of stress-activated protein kinases in MKN28 human gastric cancer cells. J Cell Physiol 223: 764-770.
- Shiozaki A, Miyazaki H, Niisato N, Nakahari T, Iwasaki Y, et al. (2006) Furosemide, a blocker of Na+/K+/2Cl- cotransporter, diminishes proliferation of poorly differentiated human gastric cancer cells by affecting G0/G1 state. J Physiol Sci 56: 401-406.
- Shiozaki A, Otsuji E, Marunaka Y (2011) Intracellular chloride regulates the G(1)/S cell cycle progression in gastric cancer cells. World J Gastrointest Oncol 3: 119-122.
- Hiraoka K, Miyazaki H, Niisato N, Iwasaki Y, Kawauchi A, et al. (2010) Chloride ion modulates cell proliferation of human androgen-independent prostatic cancer cell. Cell Physiol Biochem 25: 379-388.
- Maki M, Miyazaki H, Nakajima K, Yamane J, Niisato N, et al. (2007) Chloride-dependent acceleration of cell cycle via modulation of Rb and cdc2 in osteoblastic cells. Biochem Biophys Res Commun 361: 1038-1043.
- Maki M, Miyazaki H, Niisato N, Morihara T, Marunaka Y, et al. (2009) Blockers of K+/Cl- transporter/channels diminish proliferation of osteoblastic cells. Biomed Res 30: 137-140.
- Grisolía S, Knecht E, Hernández-Yago J, Wallace R (1979) Turnover and degradation of mitochondria and their proteins. Ciba Found Symp : 167-188.
- Kawai A, Uchiyama H, Takano S, Nakamura N, Ohkuma S (2007) Autophagosome-lysosome fusion depends on the pH in acidic compartments in CHO cells. Autophagy 3: 154-157.
- Youle RJ, Narendra DP (2011) Mechanisms of mitophagy. Nat Rev Mol Cell Biol 12: 9-14.
- Di X, Zhang G, Zhang Y, Takeda K, Rosado LA, et al. (2013) Accumulation of autophagosomes in breast cancer cells induces TRAIL resistance through downregulation of surface expression of death receptors 4 and 5. Oncotarget 4: 1349-1364.
- White E (2012) Deconvoluting the context-dependent role for autophagy in cancer. Nat Rev Cancer 12: 401-410.
- Yang L, Li P, Fu S, Calay ES, Hotamisligil GS (2010) Defective hepatic autophagy in obesity promotes ER stress and causes insulin resistance. Cell Metab 11: 467-478.
- Fritz T, Niederreiter L, Adolph T, Blumberg RS, Kaser A (2011) Crohn's disease: NOD2, autophagy and ER stress converge. Gut 60: 1580-1588.
- Amaravadi RK, Yu D, Lum JJ, Bui T, Christophorou MA, et al. (2007) Autophagy inhibition enhances therapy-induced apoptosis in a Myc-induced model of lymphoma. J Clin Invest 117: 326-336.
- Guo JY, Chen HY, Mathew R, Fan J, Strohecker AM, et al. (2011) Activated Ras requires autophagy to maintain oxidative metabolism and tumorigenesis. Genes Dev 25: 460-470.
- Yang S, Wang X, Contino G, Liesa M, Sahin E, et al. (2011) Pancreatic cancers require autophagy for tumor growth. Genes Dev 25: 717-729.
- Bohley P, Seglen PO (1992) Proteases and proteolysis in the lysosome. Experientia 48: 151-157.
- Graves AR, Curran PK, Smith CL, Mindell JA (2008) The Cl-/H+ antiporter ClC-7 is the primary chloride permeation pathway in lysosomes. Nature 453: 788-792.
- Leisle L, Ludwig CF, Wagner FA, Jentsch TJ, Stauber T (2011) ClC-7 is a slowly voltage-gated 2Cl(-)/1H(+)-exchanger and requires Ostm1 for transport activity. EMBO J 30: 2140-2152.
- Ishida Y, Nayak S, Mindell JA, Grabe M (2013) A model of lysosomal pH regulation. J Gen Physiol 141: 705-720.
- Weinert S, Jabs S, Supanchart C, Schweizer M, Gimber N, et al. (2010) Lysosomal pathology and osteopetrosis upon loss of H+-driven lysosomal Cl- accumulation. Science 328: 1401-1403.
- Lange PF, Wartosch L, Jentsch TJ, Fuhrmann JC (2006) ClC-7 requires Ostm1 as a beta-subunit to support bone resorption and lysosomal function. Nature 440: 220-223.
- Hosogi S, Miyazaki H, Kusuzaki K, Niisato N, Marunaka Y (2013) Cl- channels/transporters as new targets for cancer therapies based on disruption of autophagy ability via modification of lysosome acidification. J Physiol Sci 63: S139.
--